Article by Paul Martz
Geology – April 2005 – Colorado Central Magazine
“We don’t have earthquakes here do we?” is a question I’ve been asked a number of times over the years — although, I’ll confess, it does seem to come up more frequently at functions where alcohol is served.
As I’ve matured I’ve learned to temper my response to something on the order of “Well, in my opinion we’re just lucky Salida isn’t a pile of bricks.”
Not to put too fine a point on our topographic situation, we are more or less surrounded by mountain ranges here in Chaffee County and earthquakes are one result of the geologic faults that uplifted them. Most, if not all of our mountains are the result of repeated motion along zones of weakness in the earth’s crust, called faults. The ultimate forces causing that uplift are varied and in many instances poorly understood. The bottom line, however, is that whatever those forces may be, we’ve got them in abundance and they aren’t dead yet, only sleeping.
The object here is to explain what faulting is, how it is manifested generally, and more specifically what kinds of failure have been observed here in Central Colorado. The first part is easy. A fault is a break with motion in the rocks that make up the earth’s surface.
There are many ways that these breaks displace the rocks, and that is a key point. Motion has to occur, otherwise the break is only a fracture, not a fault. Once we figure out what kind of motion it was, we can give the structure a name to identify it. Out on the outcrop, there can be a lot of discussion about what form that motion has taken, and sometimes even if there has been any motion. To be humane to the non-geologist readers, I won’t go any farther down that path.
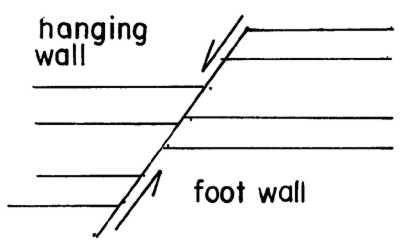
A “normal” fault in geologic parlance is one with the type of displacement shown in Figure 1. The angle of the surface along which the motion occurs is either dipping (slanting) away from the up thrown side, or vertical. One side moves vertically relative to the other, with no horizontal component. This type of fault is by far the most common form of movement one encounters when doing geologic mapping. Can it trigger a tsunami? Yes.
In the early ’90s only a few miles off the northern coast of New Guinea a shallow earthquake along a normal fault created a large tsunami. That wave came ashore so rapidly after the event that even with the type of early warning system now being discussed for the Indian Ocean, the people living along that coast would have had no chance to escape.
One major difference between Sumatra and the New Guinea event was that the latter was felt strongly on land, but it was mere minutes before the wave struck. More than ten thousand people died in New Guinea. But because the damage was confined to a remote area with no western tourists (although I do seem to remember several missionaries were killed), it received little coverage — even after the scale of the devastation became apparent.
The scariest film footage of a tsunami that I have ever seen was taken at old Valdez, Alaska from the deck of a ship that was in port at the time. The floor of the ocean on the land side suddenly sank along a normal fault. The photographer captured an image of that magic moment when the upthrown edge of the ocean was exposed before that water crashed onto shore. I’ve often wondered how the photographer and his camera survived.
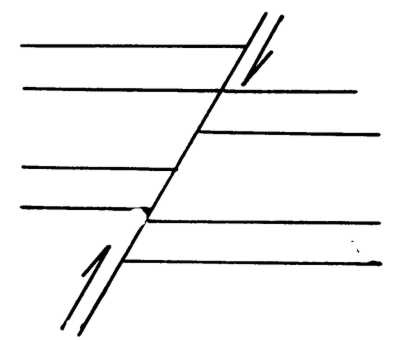
“Reverse” faults are similar to normal faults except their motion has exactly the opposite displacement. The side “beneath” the fault plane moves down rather than up, relative to the opposite side. In miners parlance, the hanging wall rises and the footwall sinks. Figure 2 illustrates the motion involved and indicates which side of the structure is the hanging wall and which side is the footwall; you stand on the footwall side.
The third type of faulting, illustrated in Figure 3, is strike-slip faulting. In this type of breakage, the main displacement is horizontal. The Sumatra quake moved the island about 100 feet to the southeast. The San Andreas fault in California, which caused the great San Francisco earthquake, is the most famous example, but hardly the only one.
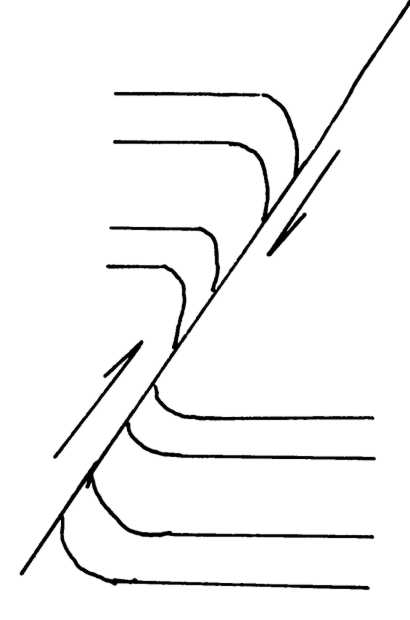
When the Rocky Mountain Arsenal began injecting toxic waste down a deep disposal well outside Denver in the early 1960s, a series of quakes with a strike-slip motion were initiated as a result. The U.S. Geological Survey correlated the pumping rate with the frequency and strength of the resulting quakes, and history was made. It was proved that man could cause an earthquake. Perhaps even more importantly, another government agency actually responded to the facts and quit pumping.
The point that usually gets overlooked about that incident, even among geologists, is that the crustal rocks of the Denver Basin had to have been under stress prior to the pumping. The injected fluids lubricated the fault zone into which the well had been drilled, but in and of themselves they would not have caused any motion to occur. The stress field that caused the motion existed previously and probably still remains. Friction between the rocks on either side of the fault was keeping movement from happening and the liquids reduced that element, which allows the pressures on opposite sides of the fault to create motion.
A sort of hybrid fault, that is actually quite common, is dip-slip motion in which both vertical and horizontal vectors result. A lot of treasure hunting articles mention famous “lost lodes,” which can be the result of dip-slip faulting (or bad treasure maps and careless prospectors who map their claims inadequately).
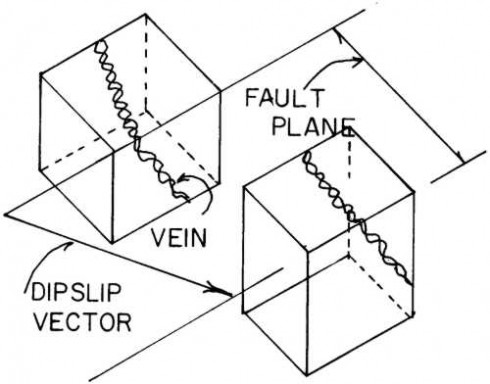
In the case of geologically lost lodes, the miners come to the abrupt end of a vein and can’t find the “lost” piece on the other side of a fault because dip-slip faulting moves things in unexpected directions.
Every time I have to figure out where to drill a test hole in that circumstance I have to get out the structural geology text, and cookbook the solution or more usually the solutions, to where that offset piece might have ended up. Given that ore deposits are geologic abnormalities themselves, and that they usually occur where the geology gets difficult to begin with, there is always more than one possibility.
In the Leadville District there are structural zones where all three of these types of motion have occurred. The often considerable expense involved to solve the puzzle of finding mineral deposits under such circumstances, especially before the invention of core drilling, was driven by the economics of high grade silver. Just because the most recent motion on a particular zone of weakness was “normal,” doesn’t mean that the first breakage, or the next one to come, will have the same type of relative displacement. When a rift turns into an arch, all the normal faults become reverse faults, or even thrusts when the center is pushed up. This is the case along a twenty plus mile stretch of the Little Belt Mountains in Montana, and may be the situation in the Howard and Coaldale areas.
Figure 5 is a thrust fault, defined by horizontal displacement of the rocks on one side over the rocks on the other. The example shown is a fold that kept on going until the rocks on one side of the axis broke, which allowed the subsequent displacement to happen. I can’t think of an example of an earthquake that was caused by motion on a thrust fault. However in central Nevada there are large parts of what was formerly Utah that slid over their western neighbor, and I have a difficult time visualizing that happening without some very significant earthquakes occurring, too.
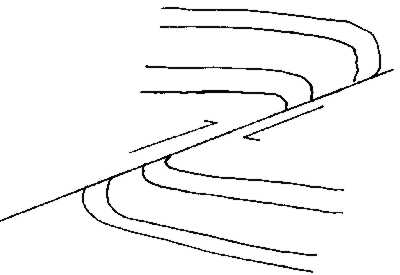
In the 1700s a large tsunami struck Japan. The quake that started it is on a fault off the Oregon and Washington coasts that is still active; it results from the westward movement of North America over the Pacific plate.
Because geologists love to split hairs — to the point of jingoism as far as I’m concerned — there are a whole slew of other fault “types” which I won’t discuss because I haven’t seen any examples of them locally.
OUR SITUATION IN Central Colorado is quite unusual because nearly all of the mountain ranges surrounding Central World Headquarters have preCambrian crystalline bedrock exposed at the bottom of the pile of subsequently deposited layers. What has been clear for a number of years is that those older rocks contain very significant faults that predate all of the subsequent events.
Not only has motion occurred along those zones of weakness many times in the past, but each time our local area is subjected to new stress, those old structures influence how that stress is distributed. Imagine a bunch of different sized blocks of wood floating in a barrel of water. Each time something disturbs the barrel, the blocks move in various ways: some move freely; some are restricted by the presence of other blocks; some get impeded by the edge of the barrel. That’s pretty much what’s going on beneath our feet around here.
The cause of the stress being placed on the crustal rocks lies deeper down in the mantle, which is a layer of plastic material, hot enough that it can turn to liquid and become magma any time the overlying pressure is released. We have had a very simplified model of the mantle for quite a long time, and I personally think it is going to be a lot longer before our understanding of it improves very much.
Having said that, it appears that currents flow within the mantle, and those currents subsequently result in stress being put on the layer of solid ground that makes up the crust. Plate tectonic activity is one form of that resulting motion, but its implications locally are beyond the scope of this article.
Those vast moving plates that they taught you about in high school (which resulted in the formation of continents and the uplift of the Himalayas) generally don’t apply in Central Colorado, because we dwell on the middle of a plate.
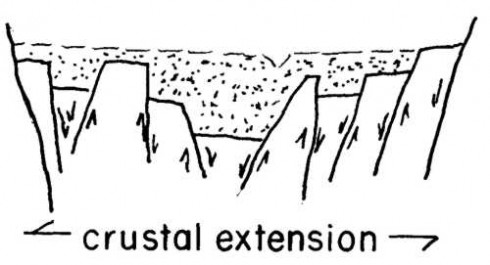
But there’s one notable exception. The Rio Grande Rift stretches from the Gulf of Mexico to Jamestown, Colorado. The rift is a zone of weakness along which the central portion of the North American continent is splitting apart, half headed east and half moving west. The resulting separation has generated large scale normal and reverse faulting, individual structures having thousands of feet of relative vertical motion and miles of failure on a single zone of weakness. Figure 6 illustrates the type of faulting present in Chaffee County resulting from the Rio Grande Rift.
Within the Rift locally, I could pay you fifty bucks every time we walk a quarter mile and don’t cross a fault — without losing anything. Imagine a sheet of glass dropped on a concrete slab and you get some idea of how ubiquitous these structures are. Not all of them are significant individually, but they can add up to hundreds of feet of vertical displacement over a relatively short horizontal distance.
When you travel along Highway 50 toward Poncha Springs, you pass by a structure that is intimately related to the uplift of the Sangre De Christo Mountains. Over a distance of tens of miles, a single plane of failure changes into zones where there are several fault strands, and then back to a single plane. On the east end, both normal and reverse faulting occurs. By the time it reaches Poncha Springs, the motion is both dip-slip and strike slip on which creep, or ultra slow motion, is occurring today.
This structure is part of a much larger zone of weakness that crosses the country, from North Carolina to California. It was recognized by Allen Hyle of the U.S.G.S. as a major crustal fracture, which he dubbed the 39th Parallel Lineament.
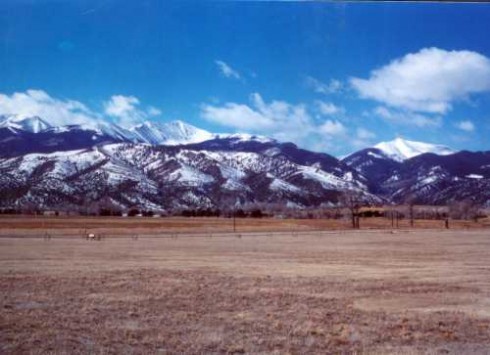
(Note how Poncha Springs sits in the area where the Rio Grande Riff and the 39th Parallel Lineament cross? Clearly, I don’t call Poncha the “Cross Hairs of the Rockies” just to annoy the Town Council, which prefers “Crossroads of the Rockies.”)
Down the Canyon to the east of Salida is another large zone of failure that is related to the development of the Arkansas Hills.
The Colorado State Geological Survey has in the last few years undertaken a program to identify which of those faults has had geologically recent motion. When that program began there were less than thirty faults that could be shown to have had motion in the last one million years. That total is now approaching one hundred, and will undoubtedly go much higher.
There are a number of structures in Chaffee county that ought to be on it, but aren’t because they have not been formally mapped, and their recent displacement has not yet been demonstrated. However, their effect on the local land surface is readily apparent.
Many of the flat surfaces that are obvious to anyone driving the highways of this area – such as Shavano Mesa which stretches from Mt. Shavano all the way to Salida — have been identified as a variety of geologic structures. And in every instance I’ve examined, these surfaces are “supported” and stabilized against erosion by caliche, which is an accumulation of calcium carbonate and sulfates, which cement the subsurface together.
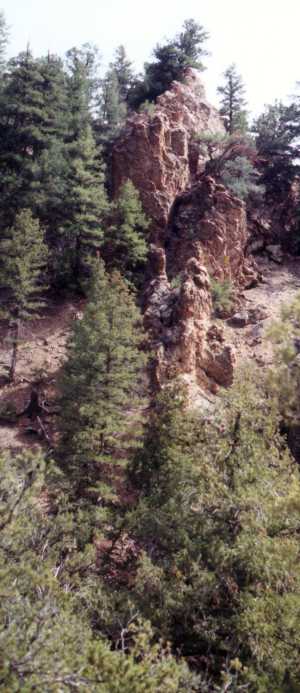
Anyone who has had a house foundation excavated, or a septic system dug on these flats, may have noticed the white coating on the material being removed. I’ve even seen it on boulders exposed in basements in downtown Salida.
Caliche is formed when a large amount of moisture falls and sinks into the ground, and then a long evaporative interval happens. Salts dissolved from the surface soil, percolate down with the water, and remain behind after evaporation to form that familiar coating. What is of particular interest about this accumulation, is that it formed on a glacial flood deposit that happened either 90 or 150 thousand years ago, depending on the scientific interpretation.
This means that at the oldest, the caliche deposits were left less than150 thousand years ago, or yesterday in geologic terms. Examination of the edges of the various surfaces today, shows them all to be under erosional attack by the present cycle of weathering. That indicates that the caliche is probably not still forming under present weather conditions. Further, when the caliche formed it appears likely that the surface in this region was as flat as the floor of the San Luis Valley today.
If one imagines these various surfaces back in a single plane, the extent of the faulting that has occurred is readily apparent. In many places, faults cut across boulder-filled stream courses of glacial outwash. This is an indication that these structures have been around for awhile.
Out in the middle of the Valley, away from the influence of the Rio Grande Rift, the offsets in the caliche-supported surface are clearly caused by the bobbling of the preCambrian basement rocks that lie beneath the soft, crumbly, easily eroded sediments that fill it.
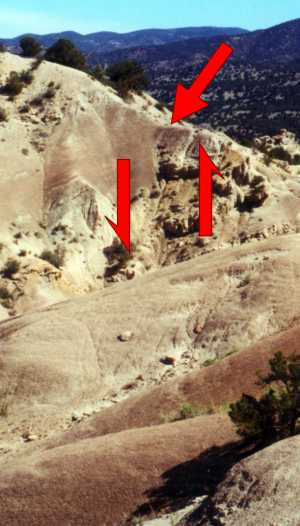
Think about those blocks of wood floating in the barrel again, and remember: “We’re just lucky Salida is not a pile of bricks.”
Paul Martz is a consulting geologist who has been fired by several Fortune 500 companies. He also owns and operates Shavano Glass in Poncha Springs.